At Interface Fluidics, we view microfluidic fluid testing as a crucial component in managing operations. The workflow highlighted here underscores the importance of screening and monitoring fluid performance as a part of an iterative process. This approach aims to enhance the efficiency of field operations by providing studies of realistic fluid-fluid interactions.
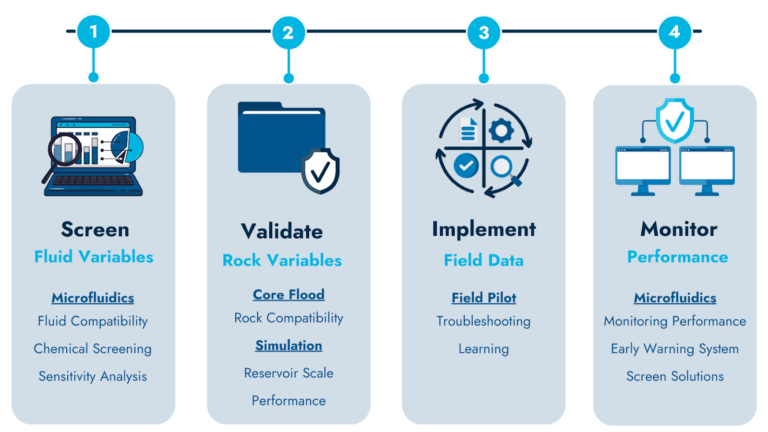
Commonly, there are a variety of tools used to help better manage subsurface oil recovery operations, one of which is reservoir simulation. The potential of incorporating datasets obtained from microfluidic testing into reservoir modelling and simulation is yet to be fully explored and it is not a routine process. This exciting frontier promises to significantly enhance the accuracy and precision of reservoir simulation, a prospect we delve into in this article.
Reservoir simulation, an essential aspect of reservoir engineering, utilizes computer models to mimic and simulate fluid flow through porous media. It enables a better understanding of reservoir operations, helping operators mitigate risks, enhance production rates, and reduce costs by identifying opportunities for increased oil production or operational savings. Serving as a valuable tool for system analysis, reservoir simulations generate numerical models of geological formations, flow equations, and fluid properties for reserve estimation, production history matching, and reservoir forecasts. The highest value of reservoir simulation lies in evaluating different field management strategies to define an optimal approach, typically resulting in higher reservoir performance along with more favourable operational and environmental outcomes. Traditionally, reservoir simulation has primarily focused on subsurface flow, modelling fluid transport from reservoir pores to the wellbore. However, there is a growing trend towards integrating subsurface flow with surface facilities in reservoir models via various coupling methods. This integration extends the scope of fluid testing characterization to model flow assurance problems as well.
The effectiveness of simulation hinges on the quality of input data and assumptions. Fluid behaviour description plays a crucial role in three stages of simulation modelling:
- during reservoir model setup, where PVT descriptions impact reserve estimations and mobility;
- in formation damage modelling, where unfavourable fluid interaction effects, such as scaling, waxing out, asphaltene precipitation, and emulsion plugging might occur and significantly affect fluid dynamics; and
- in enhanced oil recovery (EOR) processes, where realistic fluid-fluid interactions are vital, particularly in foam, surfactant, polymer, and miscible flooding scenarios.
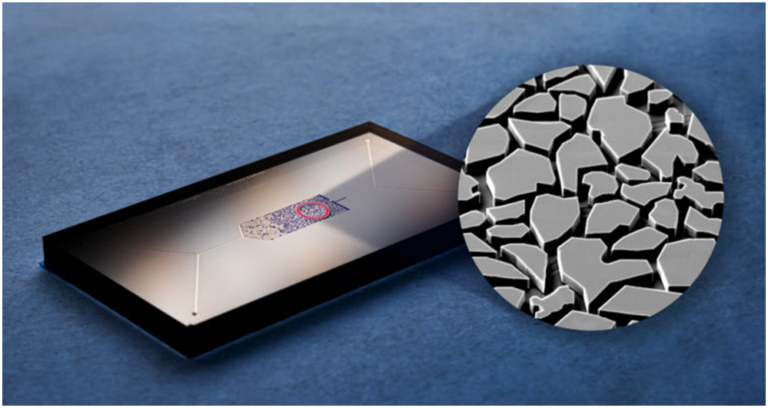
Now, let’s explore where microfluidics meets simulation modelling. For the simulation model setup, the pressure, volume, temperature (PVT) fluid descriptions can be directly obtained from microfluidic PVT analysis. Interface Fluidics routinely provides phase behaviour measurements of black oils and retrograde gas condensates via microfluidic testing, efficiently delivering accurate measurements of saturation pressures and phase volumes, with the added advantage of potential deployment at operational sites to enhance accessibility to PVT data in the energy industry [2].
Microfluidic studies on multi-gas-oil ratio (multi-GOR) can enhance the formulation of Black Oil Fluid by providing experimental insights into oil behaviour as gas leaves the solution during production. This approach also helps mitigate risks associated with initial fluid-in-place definitions when reservoir fluid samples are inaccessible. Interface Fluidics’ high-pressure, high-temperature microfluidic approach for oil swelling, with a gas-in-oil slug generator, is especially relevant for gas injection EOR processes. Our method generates comprehensive data across both bubble and dew points of reservoir oil and injection gas phases [3].
There are reservoirs simple enough to be modelled without added complexity. However, in cases where fluids are prone to create organic precipitants, scales and minerals, emulsions, and other forms of formation damage, the accuracy of these predictive models can be uncertain and questionable. The microfluidic platform accurately evaluates fluid compatibility, organic solid onset conditions, and deposition and emulsification characteristics at reservoir pressures and temperatures. The formation damage conditions and dynamics at relevant reservoir confinement can be incorporated into the reservoir simulation within nodes of solid mobility modifiers, asphaltene precipitation and viscosity effects, depending on which commercial simulator is used and its extensions.
For the formation damage testing microfluidic measurements, such as wax appearance/disappearance temperature, emulsification, asphaltene onset pressure, or even tailored asphaltene precipitation conditions in gas injection scenarios, can be directly incorporated into the simulation as phase change triggers. Interface Fluidics has developed methods to test and probe such conditions in a systematic manner with a high degree of accuracy. An example of a comprehensive test that evaluates effects of solvents on asphaltene deposition, emulsion formation, and steam chamber growth is a Steam-Assisted Gravity Drainage (SAGD) test among others. Near-wellbore plugging can be characterized by the decline in injectivity or productivity index that is derived experimentally, rather than being superficially imposed in the simulation.
EOR modelling can benefit from additional insight and information from fluid-fluid interaction studies. Foam, surfactant, polymer, and solvent flooding can be performed directly on microfluidic platforms at reservoir conditions, with the obtained results incorporated into the reservoir simulation models. A real-life example where Interface Fluidics’ polymer EOR study helped an operator to reduce polymer concentration by 60% without adverse effect on oil production and also resulted in tuning water/oil relative permeabilities for numerical modelling for better-informed decision making. [4][5]
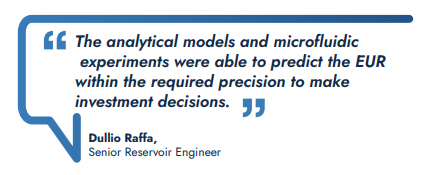
Some other examples of nodes that can help model the EOR processes are foam decay effects, mobility reductions as a function of oil/water saturations, and chemical wettability modifications. For CO2 gas injection EORs, for example, Interface Fluidics technology enables rapid testing of a wide range of gas compositions and impurities to determine minimum miscibility pressure (MMP), with unprecedented advantages over the conventional slim tube method in speed, accuracy, and sample volume [6]. Gas trapping hysteresis during the Carbon Capture and Sequestration (CCS) injections is another parameter that can be directly provided by the microfluidic testing enabled by the optical access into the porous media.
Knowing when to incorporate additional details into the reservoir simulation models and which effects to prioritize when fine-tuning the reservoir model is critical. Experienced reservoir simulation engineers, armed with specific reservoir data and production insights, can make better decisions navigating this course when equipped with more realistic fluid behaviour and interaction data. In this regard, integrating microfluidics with reservoir simulation enables informed decisions, paving the way for optimized reservoir management strategies and operational costs. Since reservoir simulation is based on the interplay between many uncertain parameters, improving the certainties by employing accurate reservoir-relevant, fluid-fluid interactions can reduce the associated risks.
For any of the tests mentioned, whether standard or customized, we are happy to discuss your specific problem and design a detailed test plan to help you find solutions.
[1] C.C. Mattax and J.R. Kyte, “Ever see a water flood?,” Oil & Gas Journal 59 (1961): 115–28, https://www.physics.purdue.edu/rockphys/docs/publications/micro-model/Mattax.pdf
[2] A Novel Method to Measure the Phase Behavior of Black Oils: Saturation Pressure and Liquid-Vapor Fractions | SPE Annual Technical Conference and Exhibition | OnePetro
[3] A Novel On-Chip Oil Swelling Measurement Platform for Gas Injection EOR Processes | SPE Improved Oil Recovery Conference | OnePetro
[4] Microfluidic-Based Optimization of Polymer flooding for Heavy Oil Recovery | SPE Canadian Energy Technology Conference | OnePetro
[5] Learnings from the Planning and Execution of a Heavy Oil Polymer Flood Pilot in North Saskatchewan, Canada | SPE Canadian Energy Technology Conference | OnePetro
[6] Large-Scale High-Throughput Sensitivity Analysis of CO2 MMP to Optimize Gas Injection EOR Processes | Abu Dhabi International Petroleum Exhibition and Conference | OnePetro